Use VCE Exam Simulator to open VCE files
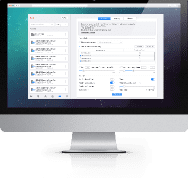
HPE6-A73 HP Practice Test Questions and Exam Dumps
Question 1
Which statement is correct regarding ACLs and TCAM usage?
A. Applying an ACL to a group of ports consumes the same resources as specific ACE entries
B. Using object groups consumes the same resources as specific ACE entries
C. Compression is automatically enabled for ASIC TCAMs on AOS-CX switches
D. Applying an ACL to a group of VLANs consumes the same resources as specific ACE entries
Answer: C
Explanation:
Access Control Lists (ACLs) are critical for controlling traffic within a network, and their efficiency depends heavily on how they are implemented in hardware, particularly in the Ternary Content-Addressable Memory (TCAM). TCAM is used in network switches for high-speed packet classification, allowing ACLs to be processed efficiently. However, because TCAM space is finite and costly in terms of hardware, efficient usage is crucial for performance and scalability.
Option C is correct because compression is automatically enabled for ASIC TCAMs on Aruba AOS-CX switches. This means that when ACLs are programmed into the TCAM, the switch software automatically optimizes and compresses the ACL entries to minimize the number of TCAM rows used. This is especially beneficial in environments where ACLs can become large and complex. The automatic compression feature helps conserve TCAM space without requiring manual optimization by the network administrator, leading to more scalable ACL deployment.
Option A is incorrect. Applying an ACL to a group of ports does not consume the same resources as applying ACLs to each port individually. When an ACL is applied to multiple ports, each application might result in separate entries in TCAM depending on the hardware implementation, which could increase resource consumption compared to applying it once globally or using shared references.
Option B is also incorrect. While object groups in ACLs (like groupings of IP addresses, protocols, or port numbers) simplify configuration and improve readability, they do not reduce the number of entries that need to be programmed into TCAM. In fact, the object groups are expanded into individual Access Control Entries (ACEs), each of which consumes TCAM space. Thus, using object groups does not inherently reduce TCAM usage.
Option D is similarly incorrect. Applying an ACL to a group of VLANs still requires creating multiple entries or references in the TCAM. The ACL has to be evaluated independently for each VLAN context, which can result in increased TCAM usage depending on how many VLANs are involved and how the hardware manages these associations.
In summary, the only option that correctly describes a method of optimizing TCAM usage with ACLs on AOS-CX switches is C, due to the automatic compression feature of the switch’s ASIC hardware. This feature directly impacts how efficiently TCAM space is utilized and allows network operators to scale ACL usage more effectively without manual intervention.
Question 2
What statement accurately describes rate limiting and egress queue shaping on AOS-CX switches?
A. Only a traffic rate and burst size can be defined for a queue
B. Limits can be defined only for broadcast and multicast traffic
C. Rate limiting and egress queue shaping can be used to restrict inbound traffic
D. Rate limiting and egress queue shaping can be applied globally
Answer: A
Explanation:
On Aruba AOS-CX switches, rate limiting and egress queue shaping are important traffic management features used to control how network traffic is forwarded from the switch. These techniques are essential for ensuring fair bandwidth distribution, preventing congestion, and maintaining the quality of service (QoS) in enterprise and data center networks.
The correct answer is A, which states that only a traffic rate and burst size can be defined for a queue. This is accurate because both rate limiting and egress queue shaping in AOS-CX rely on the configuration of parameters such as committed information rate (CIR) and burst size. These values define the maximum allowed rate at which traffic can be transmitted and how much traffic can momentarily exceed that rate (burst), respectively. This approach allows administrators to fine-tune the behavior of traffic queues on egress ports to match service-level requirements.
Egress queue shaping operates on outbound traffic and is typically applied per interface or per queue. It delays packets in a queue to ensure that the transmission rate does not exceed the configured limit. This is useful in scenarios where downstream links have lower bandwidth or when traffic must be paced to comply with service agreements.
Now let’s look at why the other options are incorrect:
Option B, which states that limits can be defined only for broadcast and multicast traffic, is not accurate. While broadcast and multicast traffic can be managed, rate limiting and shaping apply more broadly to any kind of traffic, including unicast. In fact, many QoS and traffic shaping policies are more often applied to unicast application flows to control bandwidth usage or enforce priority.
Option C suggests that rate limiting and egress queue shaping can be used to restrict inbound traffic. This is incorrect in the context of AOS-CX, where egress shaping and rate limiting are designed for outbound traffic control. Although there are ingress rate limiting mechanisms available separately (for policing), egress queue shaping specifically applies only when traffic is leaving the switch port, not when it's entering.
Option D incorrectly claims that rate limiting and queue shaping can be applied globally. In reality, these functions are applied at the port or queue level, not globally across the switch. Each interface or queue can be individually configured with specific parameters, giving precise control over traffic behavior per port or per traffic class.
To summarize, AOS-CX switches provide granular control over egress traffic using shaping and rate limiting based on defined traffic rates and burst sizes. This functionality is part of a comprehensive QoS system designed to optimize performance and enforce policy. Therefore, the only correct option is A, as it accurately reflects how shaping is configured on a per-queue basis using rate and burst parameters.
Question 3
A network administrator needs to replace an antiquated access layer solution with a modular solution involving AOS-CX switches. The administrator wants to leverage virtual switching technologies. The solution needs to support high-availability with dual-control planes.
Which solution should the administrator implement?
A. AOS-CX 8325
B. AOS-CX 6300
C. AOS-CX 6400
D. AOS-CX 8400
Answer: D
Explanation:
When designing a modern, resilient access layer for an enterprise network, a key consideration is high availability, which often requires redundancy not only in hardware components but also in control planes. A dual control plane setup ensures that even if one management processor fails, the system can continue functioning with minimal disruption. This is particularly critical in environments where downtime is unacceptable.
Among the options provided, AOS-CX 8400 is the only platform that is both modular and supports high availability with dual-control planes. It is designed for enterprise core and aggregation layers, but it can also be used in high-performance access layers where redundancy, scalability, and advanced features are required. The AOS-CX 8400 series offers a chassis-based architecture with support for redundant management modules, power supplies, and fan trays. This platform is purpose-built for virtual switching technologies and high-availability needs.
Option A, the AOS-CX 8325, is a fixed-form factor switch. While it does support virtual switching frameworks such as VSX (Virtual Switching Extension), it lacks a modular design and does not offer dual control planes. This makes it less suitable for the scenario where the requirement explicitly calls for a modular and highly available architecture.
Option B, the AOS-CX 6300, is also a fixed configuration switch. It supports stacking and can be used effectively at the access layer, but it does not meet the requirement of a modular solution or dual control planes. It's a strong option for many access layer deployments, but not when high availability at the control plane level is a priority.
Option C, the AOS-CX 6400, is indeed modular and suitable for access or aggregation layers. However, it does not support dual control planes. The 6400 series typically uses a single management module, making it a less suitable option where uninterrupted control plane functionality is required.
In contrast, the AOS-CX 8400 was built with high availability and modular scalability in mind. Its architecture enables critical features such as hitless software upgrades, fault isolation, and non-stop forwarding, making it the ideal candidate for the administrator’s requirements.
Therefore, the best solution that aligns with the need for a modular platform, virtual switching, and dual control planes is D, the AOS-CX 8400.
Question 4
A company has enabled 802.1X authentication on its AOS-CX access switches using two ClearPass servers for AAA. Each switch includes a configuration line that reads: radius-server tracking user-name monitor password plaintext aruba123.
What is the purpose of this command?
A. Implement replay protection for AAA messages
B. Define the account to implement downloadable user roles
C. Speed up the AAA authentication process
D. Define the account to implement change of authorization
Answer: D
Explanation:
In AOS-CX switches, the command radius-server tracking user-name monitor password plaintext aruba123 is used to configure an account that enables RADIUS server tracking functionality. Specifically, this tracking mechanism is required for the switch to perform Change of Authorization (CoA) operations successfully with RADIUS servers like Aruba ClearPass.
Change of Authorization (CoA) is a feature used in 802.1X environments to dynamically alter a user's session after the initial authentication has completed. This could include changing the VLAN assignment, applying a new policy, or even disconnecting a user session when necessary. In order for the switch to initiate such a change with the RADIUS server, it must send RADIUS requests on its own behalf—these requests require an authenticated identity to be accepted by the RADIUS server. That’s exactly what the radius-server tracking command provides.
Option D is therefore correct because this command defines a user account (in this case, "monitor") with a password ("aruba123") that the switch can use to perform CoA requests to the RADIUS server. These requests are out-of-band (i.e., initiated by the switch rather than by the end client), and they require authentication—hence the need to define credentials.
Now let's examine why the other options are incorrect:
Option A, which claims the configuration is for implementing replay protection for AAA messages, is incorrect. Replay protection typically involves mechanisms like timestamps and nonces, not static user credentials for server communication. The radius-server tracking command has no role in preventing replay attacks; it is used solely for switch authentication toward the RADIUS server during specific operations like CoA.
Option B, suggesting the account is used to implement downloadable user roles, is not accurate. Downloadable user roles are usually delivered by the RADIUS server as part of the access-accept response after user authentication. While CoA may alter these roles post-authentication, the account configured with the radius-server tracking command is not used to receive downloadable roles but to initiate CoA messages.
Option C, stating that this command speeds up the AAA authentication process, is misleading. The command does not influence the timing or efficiency of the actual 802.1X authentication or RADIUS exchanges. Instead, it is specifically associated with tracking server availability and performing CoA operations, which are secondary and administrative in nature.
In summary, the radius-server tracking user-name monitor password plaintext aruba123 command is used to allow the AOS-CX switch to authenticate itself to the RADIUS server when performing Change of Authorization tasks. This is critical in dynamic environments where user sessions may need to be modified or terminated without restarting the entire authentication process. Thus, the correct answer is D.
Question 5
A company has an existing wireless solution involving Aruba APs and Mobility controllers running 8.4 code. The solution leverages a third-party AAA solution. The company is replacing existing access switches with AOS-CX 6300 and 6400 switches. The company wants to leverage the same security and firewall policies for both wired and wireless traffic.
Which solution should the company implement?
A. RADIUS dynamic authorization
B. Downloadable user roles
C. IPSec
D. User-based tunneling
Answer: D
Explanation:
In a network architecture that includes both wired and wireless clients, maintaining consistent security enforcement—particularly firewall policies and access control—is a common challenge. Aruba provides a unique feature called User-Based Tunneling (UBT), which solves this issue by allowing traffic from wired clients to be tunneled to a centralized Mobility Controller. This is the same way Aruba wireless clients are handled, enabling the network to apply consistent role-based access policies across both wired and wireless users.
In this scenario, the company is using Aruba APs and Mobility Controllers running 8.4 code, and is introducing AOS-CX 6300 and 6400 switches. These AOS-CX switches support User-Based Tunneling, which allows the wired switch to tunnel user traffic to the Mobility Controller, where policies such as firewall rules, VLAN assignments, bandwidth contracts, and other access controls can be applied consistently across both wireless and wired endpoints. This integration makes policy management much more unified and scalable.
Option A, RADIUS dynamic authorization (also known as CoA—Change of Authorization), allows for real-time policy enforcement and changes, such as disconnecting a user or changing VLANs. However, this is a mechanism for changing policy, not for enforcing consistent firewall or security policies across platforms. It does not centralize policy enforcement the way tunneling to a controller does.
Option B, downloadable user roles (DUR), is an Aruba feature that allows role-based access policies to be dynamically pushed to a switch upon user authentication. While DUR provides local policy enforcement at the switch level and does offer flexibility, it still does not offer the full policy consistency and centralization that UBT provides via the controller, especially for complex firewall rules.
Option C, IPSec, is a tunneling and encryption protocol used to secure traffic at the IP layer. While secure, it is not designed for unified policy enforcement across wired and wireless users in the way required here. It’s also not the typical method Aruba uses to extend controller-based policies to the wired edge.
User-Based Tunneling is the most appropriate and powerful solution here. It enables wired clients on AOS-CX switches to be treated just like wireless clients, ensuring a consistent experience and centralized policy enforcement via the Mobility Controller, which already handles the wireless environment. This also simplifies administration and compliance by consolidating security rules in a single location.
Thus, the correct solution for applying the same firewall and security policies across wired and wireless traffic is D, User-Based Tunneling.
Question 6
Which command sequence correctly identifies a VLAN as a voice VLAN on an AOS-CX switch?
A. Switch(config)# port-access lldp-group <LLDP-group-name>
Switch(config-lldp-group)# vlan <VLAN-ID>
B. Switch(config)# port-access role <role-name>
Switch(config-pa-role)# vlan access <VLAN-ID>
C. Switch(config)# vlan <VLAN-ID>
Switch(config-vlan-<VLAN-ID>)# voice
D. Switch(config)# vlan <VLAN-ID> voice
Answer: C
Explanation:
In Aruba AOS-CX switches, designating a VLAN as a voice VLAN is essential when setting up ports to support IP phones using Voice over IP (VoIP). This configuration ensures that the switch handles voice traffic with appropriate priority, isolation, and, where applicable, integration with LLDP-MED for dynamic VLAN assignment. Voice VLANs typically enable phones to automatically receive the correct VLAN information from the switch when they connect.
The correct way to identify a VLAN as a voice VLAN is shown in option C:
Switch(config)# vlan <VLAN-ID>
Switch(config-vlan-<VLAN-ID>)# voice
This command marks the specified VLAN as a voice VLAN within the switch configuration. It does not assign the VLAN to a port or an interface directly. Instead, it designates the VLAN as being used for voice traffic. This is critical for environments where LLDP-MED is used to dynamically inform VoIP devices (like IP phones) about the appropriate voice VLAN ID.
Here’s a breakdown of why the other options are incorrect:
Option A involves configuring an LLDP group and associating a VLAN with it. While LLDP-MED can be used to communicate the voice VLAN to phones, simply linking a VLAN in an LLDP group does not by itself designate the VLAN as a voice VLAN. It is part of a broader configuration and does not set the global VLAN voice designation.
Option B creates a port-access role and sets a VLAN under that role. While this might be part of a dynamic access policy (such as for 802.1X authenticated clients), it does not mark the VLAN as a voice VLAN at the global VLAN configuration level. Port-access roles manage how endpoints are assigned to VLANs and what traffic policies they follow, but the voice designation is not part of this configuration.
Option D is syntactically incorrect. The command Switch(config)# vlan <VLAN-ID> voice attempts to apply the voice keyword in the wrong context and format. AOS-CX requires the voice configuration to be executed within the VLAN configuration context, not inline with the VLAN creation command.
Designating a VLAN as a voice VLAN helps in ensuring that when a device such as an IP phone connects to a port (often with the aid of LLDP-MED), the switch recognizes and assigns the appropriate VLAN and applies any QoS or priority settings associated with voice traffic.
In conclusion, only option C uses the correct syntax and context to mark a VLAN for voice traffic on AOS-CX switches. This step is essential for any enterprise voice deployment using VoIP, particularly when relying on automated provisioning and quality-of-service features.
Question 7
An administrator will be replacing a campus switching infrastructure with AOS-CX switches that support VSX capabilities. The campus involves a core, as well as multiple access layers.
Which feature should the administrator implement to allow both VSX-capable core switches to process traffic sent to the default gateway in the campus VLANs?
A. VRF
B. VRRP
C. IP helper
D. Active gateway
Answer: D
Explanation:
In a campus network using AOS-CX switches with VSX (Virtual Switching Extension), the goal is to ensure high availability and load balancing across the core switches. A critical component of this design is the ability to have both VSX peer switches function as default gateways for VLANs simultaneously, ensuring seamless failover and consistent traffic processing.
The feature that enables this is called Active Gateway. With Active Gateway, both VSX switches are configured with the same virtual IP and MAC address for the default gateway of a VLAN. Unlike traditional VRRP (Virtual Router Redundancy Protocol), which has one active router and one standby router, Active Gateway allows both core switches to be active and forward traffic simultaneously, providing better load sharing and redundancy.
Option A, VRF (Virtual Routing and Forwarding), is a technology used to create multiple isolated routing tables on the same physical switch. While powerful for network segmentation and multi-tenancy, VRF is not related to the default gateway redundancy or traffic processing in this context.
Option B, VRRP, is a standard protocol used to provide high availability for default gateways. However, in VRRP, only one router (or switch) is active at any time; the other remains on standby. This does not allow for both VSX switches to forward traffic simultaneously. Additionally, VRRP is not the preferred or optimized method in VSX designs, where Active Gateway is purpose-built for this functionality.
Option C, IP helper, is used to forward broadcast requests (typically DHCP) to a remote server, allowing clients in one subnet to receive DHCP addresses from a server in another subnet. It does not address the need for default gateway processing or load balancing across core switches.
Option D, Active Gateway, is the correct and Aruba-recommended solution for VSX environments. It ensures both core switches in the VSX pair are active participants in routing, which means they both handle client traffic sent to the default gateway. This improves resilience and efficiency in the network and aligns with best practices for campus deployments using VSX.
In summary, Active Gateway is the only feature listed that provides simultaneous active gateway functionality across both core switches in a VSX pair, enabling load balancing and high availability for VLAN default gateways. Therefore, the correct answer is D.
Question 8
Which statement correctly describes how user traffic is tunneled between AOS-CX switches and Aruba Mobility Controllers (MCs)?
A. Uses IPSec to protect the management and data traffic
B. Uses IPSec to protect the management traffic
C. Supports only port-based tunneling
D. Uses the same management protocol as Aruba APs
Answer: B
Explanation:
In Aruba networks, the tunneling of user traffic from AOS-CX switches to Aruba Mobility Controllers (MCs) is a feature that enables centralization of user traffic for policy enforcement, access control, and security. This is commonly used in Dynamic Segmentation, a key capability of Aruba’s architecture that helps unify wired and wireless user access policies.
The correct answer is B, which states that IPSec is used to protect the management traffic between the AOS-CX switch and the Mobility Controller. This is accurate and aligns with how Aruba secures the control-plane communications that establish and manage the tunnels. These management channels handle the negotiation and maintenance of GRE tunnels, user authentication status, and policy assignments.
The actual user traffic itself—once the tunnel is established—is transported using GRE (Generic Routing Encapsulation). This is done to ensure high performance and low overhead. GRE is less computationally expensive compared to IPSec for large volumes of traffic, making it ideal for user data forwarding. Therefore, while IPSec is used for management/control traffic, the data plane uses GRE tunnels.
Let’s evaluate why the other options are incorrect:
Option A, which claims that both management and data traffic are protected by IPSec, is incorrect. This is a common misconception. Aruba uses IPSec selectively—only for the control plane (i.e., the management traffic that coordinates tunnel setup, authentication, etc.). The data plane traffic uses GRE because encrypting all data traffic with IPSec would introduce significant latency and processing overhead on the switches and controllers, especially in high-throughput environments.
Option C, stating that only port-based tunneling is supported, is not accurate. Aruba’s solution supports both port-based and user-based tunneling. Port-based tunneling involves directing all traffic from a specific switch port through a GRE tunnel to the Mobility Controller. In contrast, user-based tunneling allows for dynamic assignment based on 802.1X authentication and policy decisions, enabling per-user segmentation and access control—one of the core capabilities of Aruba’s Dynamic Segmentation.
Option D, which says that the switches use the same management protocol as Aruba APs, is misleading. Aruba Access Points use CAPWAP (Control And Provisioning of Wireless Access Points) for control and management with Aruba Controllers, which is specific to wireless infrastructure. AOS-CX switches do not use CAPWAP. Instead, their communication with controllers relies on GRE tunneling for data traffic and IPSec for management traffic, implemented as part of Aruba’s dynamic segmentation and switch-role functionality. These are distinct and optimized for wired networks.
In summary, the Aruba solution separates the control and data planes, using IPSec for secure management traffic and GRE for efficient data traffic. This hybrid tunneling model balances security with performance, and option B most accurately reflects that architecture.
Question 9
An administrator is implementing a multicast solution in a multi-VLAN network. Which statement is true about the configuration of the switches in the network?
A. IGMP snooping must be enabled on all interfaces on a switch to intelligently forward traffic
B. IGMP requires join and leave messages to graft and prune multicast streams between switches
C. IGMP must be enabled on all routed interfaces where multicast traffic will traverse
D. IGMP must be enabled on all interfaces where multicast sources and receivers are connected
Answer: C
Explanation:
To properly support multicast in a network that includes multiple VLANs, it is essential to understand the roles of both IGMP (Internet Group Management Protocol) and PIM (Protocol Independent Multicast).
Multicast operates by sending traffic to multiple receivers without replicating data for each one individually. Instead, a single multicast stream is sent, and switches and routers replicate it only when necessary. IGMP is the protocol used between hosts and the first-hop router to manage multicast group memberships.
In a multi-VLAN network, multicast traffic must be routed between VLANs, because each VLAN is typically a separate subnet. This means the multicast-capable router or Layer 3 switch must participate in multicast routing. For multicast routing to function correctly, IGMP must be enabled on all routed interfaces (SVIs) where multicast traffic will enter or leave, as this is where multicast group membership reports are exchanged between the router and hosts.
Option A is incorrect because IGMP snooping is a feature of Layer 2 switches that helps control multicast traffic within a VLAN by examining IGMP messages between hosts and routers. However, it does not need to be enabled on all interfaces; it is generally enabled globally and operates on a per-VLAN basis, not per-interface.
Option B is incorrect because IGMP does not manage multicast forwarding between switches. That is the job of PIM, not IGMP. IGMP handles communication between hosts and their first-hop router, not inter-switch communication.
Option D is misleading. While IGMP messages originate from interfaces where hosts (receivers or sources) reside, IGMP is not something you enable on individual Layer 2 switch interfaces. Instead, IGMP is enabled on routed interfaces, such as VLAN interfaces or routed physical ports, on the router or Layer 3 switch that is acting as the multicast querier.
Therefore, the most accurate and correct statement is C: IGMP must be enabled on all routed interfaces where multicast traffic will traverse. This allows routers to process IGMP membership reports and manage the multicast forwarding accordingly, often in conjunction with PIM for building multicast distribution trees across the network.
Top Training Courses
SPECIAL OFFER: GET 10% OFF
This is ONE TIME OFFER
A confirmation link will be sent to this email address to verify your login. *We value your privacy. We will not rent or sell your email address.
Download Free Demo of VCE Exam Simulator
Experience Avanset VCE Exam Simulator for yourself.
Simply submit your e-mail address below to get started with our interactive software demo of your free trial.